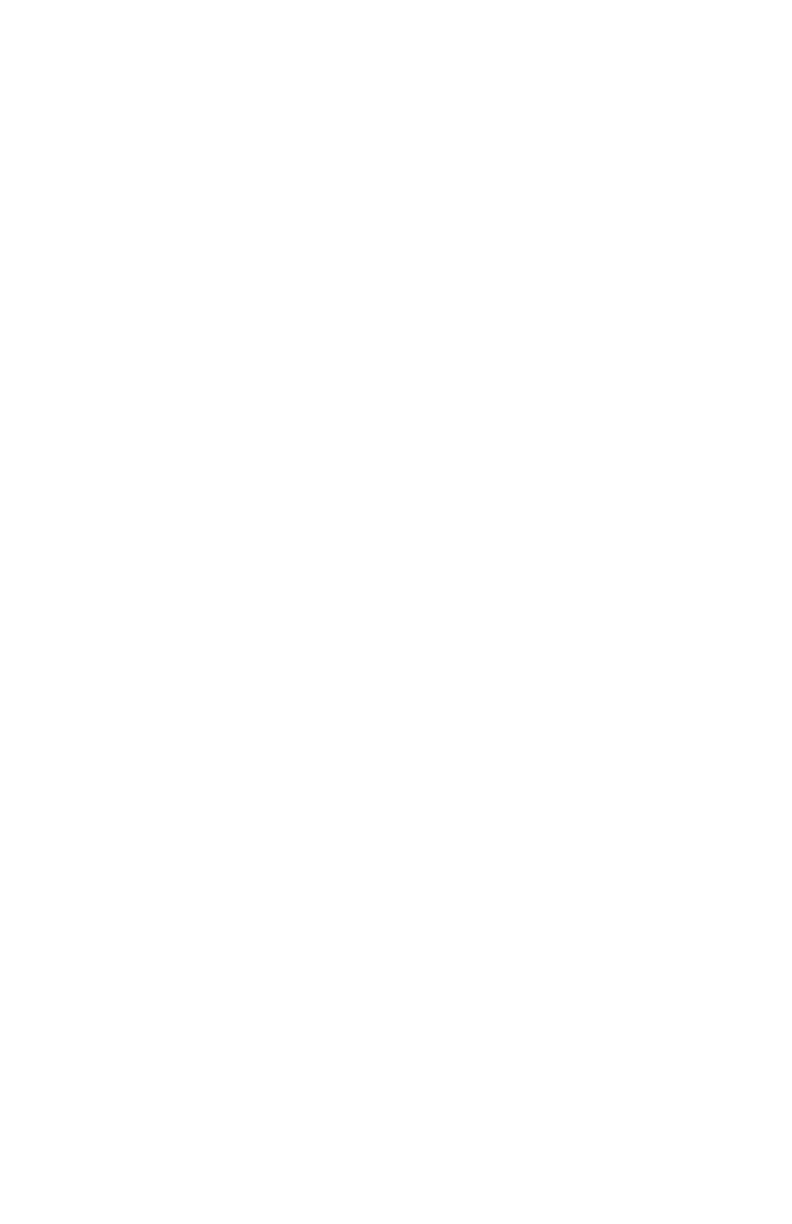
4 | SAMLEX AMERICA INC.
OWNER'S MANUAL | Index
List of Tables
Table 3.1 Battery Sizing Formulas ......................................................................9
Table 3.2 Popular Battery Sizes ........................................................................13
Table 3.3 Battery Capacity versus Rate of Discharge .......................................13
Table 3.4 Typical Cycle Life Chart ....................................................................14
Table 3.5 Absorption Voltage vs Temperature (example)...................................14
Table 4.1 Lithium Battery Prole Settings ........................................................24
Table 5.1 LED Display for Charging Stages & Faults ........................................26
Table 5.2 Push Button and Switch Descriptions ...............................................26
Table 5.3 Operational Screens .........................................................................29
Table 5.4 LCD Display Parameters ...................................................................30
Table 6.1 Recommended Wire Size ................................................................. 32
Table 6.2 Dip Switching Settings .....................................................................35
Table 6.3 Standard Battery Charging Programs ............................................... 36
Table 6.4 Temperature Compensation of Voltage Settings .............................. 38
Table 7.1 Fault Indications & Remedies ...........................................................42
List of Figures
Fig 2.1 Current (I), Voltage (V) and Power (P) Curves ........................................6
Fig 2.2 I-V Curve and Ratings of a 12V PV / Solar Panel ......................................7
Fig 3.1 Series Connection ...............................................................................10
Fig 3.2 Parallel Connection .............................................................................10
Fig 3.3 Series-Parallel Connection ...................................................................11
Fig 3.4 Temperature vs State of Charge ..........................................................15
Fig 4.1 Series Type PWM Control – PWM Frequency = 300 Hz .......................18
Fig 4.2A Normal Charging Algorithm .............................................................19
Fig 4.2B Equalization Algorithm ...................................................................... 19
Fig 5.1 EVO-30AB Layout ................................................................................25
Fig 5.2 EVO-RC-PLUS Layout ...........................................................................27
Fig 5.3 Startup Screen .....................................................................................28
Fig 5.4 EVO-RC-PLUS and EVO-30AB Menu Map ...........................................31
Fig 6.1 EVO-30AB Dimensions ........................................................................ 33
Fig 6.2 EVO-30AB Wiring Diagram ................................................................. 34
Fig 6.3 EVO-30AB with EVO-RC-PLUS Wiring Diagram ..................................39
Fig 6.4 EVO-30AB with EVO-RC-PLUS and
EVOTM Inverter/Charger Wiring Diagram .............................................40
Fig 6.5 EVO-30AB with EVOTM Inverter/Charger .............................................41
Disclaimer of Liability
UNLESS SPECIFICALLY AGREED TO IN WRITING, SAMLEX AMERICA INC.:
1. MAKES NO WARRANTY AS TO THE ACCURACY, SUFFICIENCY OR SUITABILITY OF ANY TECHNICAL OR OTHER INFORMATION
PROVIDED IN ITS MANUALS OR OTHER DOCUMENTATION.
2. ASSUMES NO RESPONSIBILITY OR LIABILITY FOR LOSSES, DAMAGES, COSTS OR EXPENSES, WHETHER SPECIAL, DIRECT,
INDIRECT, CONSEQUENTIAL OR INCIDENTAL, WHICH MIGHT ARISE OUT OF THE USE OF SUCH INFORMATION. THE USE OF
ANY SUCH INFORMATION WILL BE ENTIRELY AT THE USERS RISK.
Samlex America reserves the right to revise this document and to periodically make changes to the content
hereof without obligation or organization of such revisions or changes.
Copyright Notice/Notice of Copyright
Copyright © 2022 by Samlex America Inc. All rights reserved. Permission to copy, distribute and/or modify this
document is prohibited without express written permission by Samlex America Inc.